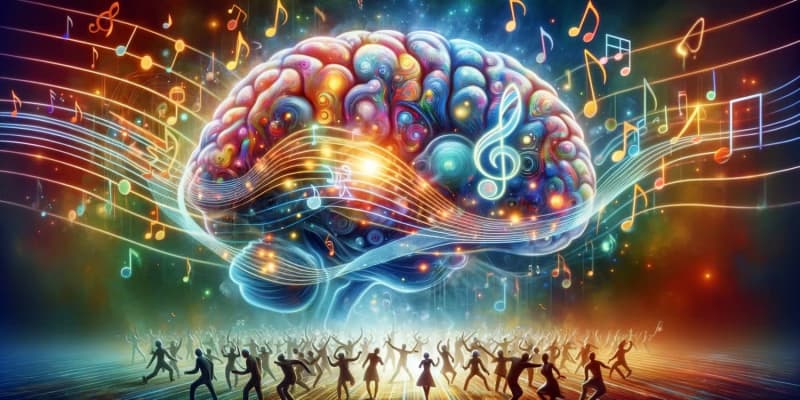
Have you ever found yourself tapping your foot or nodding your head to the rhythm of a catchy tune, seemingly without any effort? It turns out, there’s a scientific explanation behind our instinctual desire to move along with music. A recent study published in Science Advances sheds light on why certain rhythms make us want to dance more than others.
By analyzing brain activity and the sensation known as ‘groove,’ researchers discovered that a rhythm of moderate complexity triggers the highest desire to move. This desire is mirrored in our brains, particularly within the left sensorimotor cortex, hinting at a deeply intertwined relationship between motor actions and sensory processes.
Prior studies have shown that even without actual movement, the perception of rhythmic music can activate areas of the brain associated with movement, such as the premotor cortices and basal ganglia. This activation suggests a link between how we process time through movement and how we perceive music.
Building on this foundation, the authors of the new study aimed to unravel the neurophysiological underpinnings of the groove by examining how changes in rhythmic properties of music could induce motor engagement through alterations in audio-motor neural dynamics.
“In speech and music, rhythm appears to be a crucial parameter for capturing auditory sensory information. Furthermore, previous studies have implicated the motor-dedicated cortical area in time perception. Initially, we aimed to investigate the implications of these motor dynamics in auditory perception,” said Arnaud Zalta, the first author of the study and a postdoctoral fellow at ENS-PSL.
To explore these dynamics, the researchers conducted a series of experiments involving 111 participants across different settings, including online surveys, magnetoencephalography (MEG) sessions, and control tapping tasks. Participants ranged in age from 19 to 71 years, with a majority being females, and were selected without regard to their musical or dance background.
In the core of these experiments was a collection of 12 original melodies, each manipulated to vary in rhythmic predictability by adjusting the degree of syncopation. Syncopation, in this context, refers to the disruption of regular rhythm by placing accents on weak beats, creating a musical “hiccup” that challenges the listener’s temporal expectations.
For the online survey component, participants were directed to a webpage where they listened to each melody through headphones or earphones. After each melody, they rated their level of groove on a Likert scale, expressing how much they felt moved to dance. This straightforward task was designed to capture the subjective experience of groove in a controlled yet flexible online setting.
In the laboratory, the MEG experiment took a deeper dive into the neurological underpinnings of groove. Participants listened to the same set of melodies while their brain activity was recorded using MEG, a technique capable of detecting the magnetic fields generated by neural activity. This allowed the researchers to observe how different rhythms influenced brain dynamics, particularly in areas associated with movement and auditory processing.
Finally, the control tapping experiment provided a behavioral counterpart to the brain imaging data. Participants tapped along to the rhythms of the melodies on a keyboard, offering a tangible measure of their motor engagement with the music. This task complemented the MEG findings by linking the subjective feeling of groove with observable motor responses.
The researchers discovered that our desire to dance, or the feeling of groove, is most strongly elicited by melodies with a medium level of syncopation. This finding implies that rhythms which strike a balance between predictability and rhythmic complexity are the most effective in inducing the urge to dance. In other words, rhythms that are neither too simple nor too complex, but rather those that offer a moderate challenge to our anticipatory and motor systems, are most likely to get us on our feet.
The researchers further uncovered that this groove sensation is closely tied to specific patterns of brain activity. Participants exhibited a unique neural response when listening to syncopated rhythms, with the left sensorimotor cortex — a brain region involved in coordinating auditory and motor information — playing a pivotal role.
This area of the brain showed increased engagement when participants were exposed to rhythms that evoked a strong desire to move. This suggests that the left sensorimotor cortex not only processes the music we hear but also anticipates and prepares our bodies for movement, acting as a bridge between hearing a rhythm and physically responding to it.
“The brain region which is the site of the left sensorimotor cortex is currently considered to be the potential cornerstone of sensorimotor integration, essential for the perception of both music and speech. The fact that it appears in our study as necessary for ‘cooperation’ between the auditory and motor systems reinforces this hypothesis, especially as we are using natural stimuli here,” explained senior author Benjamin Morillon of Aix-Marseille Université.
Moreover, the study introduced a neurodynamic model to explain the transformation of syncopated rhythms into the subjective experience of groove. This model proposes that our brains interpret the rhythms through a network of oscillators, which then translate these rhythms into motor engagement signals. Interestingly, the degree of syncopation correlated with neural activity at a specific frequency (2 Hz), indicating that our brain’s response to music involves an interaction between auditory perception and motor preparation.
“Motor actions and sensory processes are closely intertwined to help us adapt better to our environment,” Zalta told PsyPost. “Specifically, when we listen to something, time becomes crucial as the auditory stimuli inherently impose high temporal constraints. When the auditory brain regions struggle to process temporal information accurately, motor dynamics appear to be recruited.
“Furthermore, we observed that sensorimotor regions play a mediating role between sensory auditory and motor regions. In short, it is the interplay of these three regions that gives rise to the sensation of groove.”
The research also highlighted a spectral gradient along the dorsal auditory pathways when participants listened to music. This means that as one moves from the auditory regions of the brain towards the motor areas, the dominant frequency of brain activity progressively increases. Lower frequencies were noted in regions closer to auditory processing, while higher frequencies were found as the gradient approached areas implicated in motor control.
“When we examined the cortical dynamics of the brain during our task, we observed an ascending postero-anterior gradient which was not expected,” Zalta said. “However, this gradient does not seem to be related to the sensation of groove or the level of syncopation of the stimuli. This phenomenon remains unclear.”
Regarding the long-term goals for this line of research, Zalta explained that “the dopaminergic system is closely intertwined with motor processes and has been implicated in time perception. I aim to delve deeper into investigating this neurotransmitter.”
The study, “Neural dynamics of predictive timing and motor engagement in music listening,” was authored by Arnaud Zalta, Edward W. Large, Daniele Schön, and Benjamin Morillon.